In SMARTeeSTORY, TECNALIA leads Work Package 3, which aims to develop a new approach to operational modelling, optimisation and control of buildings.
The work package aims to develop a new approach to operational modelling, optimisation, and control of buildings by creating methods for understanding and managing large amounts of data. This will result in a tool that will help researchers and organisations analyse and use data effectively. Building Performance Simulation (BPS) is a powerful tool to represent various aspects of buildings’ performance. Lets explore the BPS and discover how SMARTeeSTORY partners use BPS to turn historical buildings into climate-neutral landmarks.
What is building performance simulation?
Building Performance Simulation (BPS) represents various aspects of buildings’ performance using physic-based models. These models consider key building characteristics, such as geometry, constructive materials, heating, ventilation, and air conditioning (HVAC) systems, lighting, and occupancy. By applying mathematical equations based on physical laws, these models simulate building energy behavior under different conditions, which can be simulated using commercial tools like DesignBuilder, EnergyPlus, TRNSYS, Modelica, and APACHE.
By simulating dynamic interactions between energy and mass flows within the building and its surroundings over time, BPS models are capable of characterising energy use, thermal behaviour, and other performance aspects such as illuminance or indoor air quality. Thus, they serve as effective tools for building performance asessment for both designing new buildings and assessing existing ones.
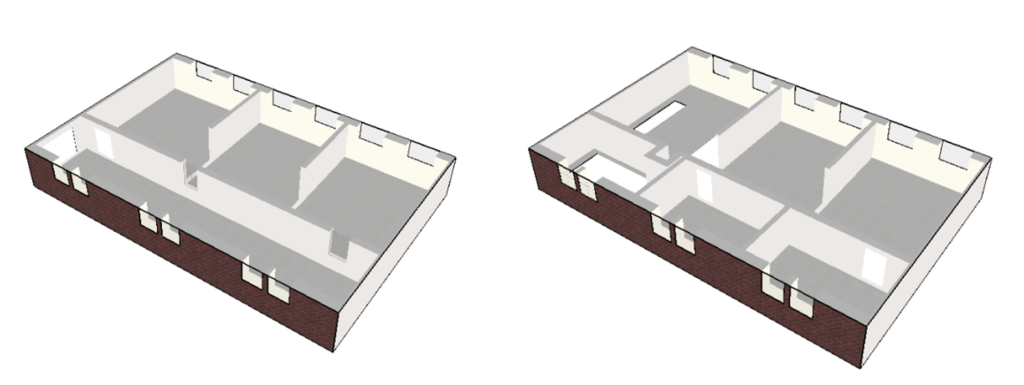
Creating a BPS model
In order to build up a physics-based model, some input parameters must be provided to the tool. These parameters represent various factors affecting internal ambient temperature and indoor environmental quality of a building. They include:
- External weather conditions
- Thermo-physical characteristics of the building envelope
- Occupancy patterns
- HVAC, DHW and lighting systems description
(generation and distribution sub-systems, and terminal units) - Operational patterns for HVAC, DHW and lighting systems
(setpoints, schedules, etc.) - Specific control strategies for HVAC, DHW and lighting systems
- Other energy transformation, transmission, and emission components
- Passive solutions such as: smart blinds, external shading, window opening, etc.
[ HVAC: Heating, ventilation, and air conditioning; DHW: Domestic hot water ]
Best practices for the data gathering process
Creating accurate BPS models involves comprehensive data gathering and a thorough understanding of the building. This is important in existing buildings where building operation in reality may not match the predicted behaviour. Key data gathering steps include:
- Assessing data availability and accuracy (geometrical, energetic, operational).
- Conduct technical visits to verify building operation and collect user behaviour data.
- Engage with maintenance and energy management teams.
- Consider new monitoring systems if needed.
When gathering data, some required information for the energy model may be incomplete. To address this lack of data the following methods are recommended:
- Building Data/Information: This includes physical structure, system characteristics, and internal gains. When real building data is lacking, consider using reference values from public databases or local building policies.
- Weather Data: when measured weather (e.g., outdoor temperature, solar radiation) is not available, consider using public databases from nearby weather stations.
After data gathering, the calibration reduces the performance gap between simulated outputs and real data. In this regard, following steps could be helpful:
- Assume Input Parameters: Document assumptions.
- Verify Simulation Results: Check if predicted operating results (e.g., temperature, humidity) are reasonable.
- Compare Energy and Demand Results: Use metered data for comparison. Assess differences between simulation and calibration data.
- Revise Assumed Input Data: Adjust assumptions to improve predicted results.
[ steps based on Option D of the International Performance Measurement and Verification Protocol: Concepts and Options for Determining Energy and Water Savings; Volume I: Revised March 2002 (nrel.gov) ]
BPS modelling for building retrofitting
BPS modelling can support various phases from the design to operation, even to test renovation solutions from the digital or physical point of view. In renovation, BPS modelling could focus on the design process and code compliance assessment or even real-time building control as part of the retrofitting.
In SMARTeeSTORY, accurate physic-based models are being developed for different purposes. At an early stage, physic-based models will generate synthetic data to calibrate the long- and short-term prediction models used in following stages. Afterwards, these models will serve for long-term assessment of building performance, to be used throughout the pre-intervention energy assessment and target setting process as well as performance assessment along the operation of SMARTeeSTORY. At last, the physic-based models will create an emulation environment to test control logics and algorithms before their deployment at real building level.
Lessons learned
From our BPS model development experience, we have identified some aspects that should be considered for anyone intending to develop these models, especially in the renovation scenario:
- Data Limitations:
- Lack of architectural and physical data leads to model inaccuracy and reduced reliability.
- Developing physics-based models is time-consuming. Communication with facility managers or knowledgeable individuals is crucial.
- When modelling small areas within a building, consider boundary conditions (e.g., surrounding rooms).
- Defining Objectives:
- Clearly define model objectives and functionalities:
- General performance assessment (e.g., HVAC sizing, envelope comparison)
- Specific element assessment or control (e.g., smart shading, strategies)
- Collaborate with partners to address specific needs (e.g., SMARTeeSTORY intervention packages).
- Clearly define model objectives and functionalities:
- Importance of Monitoring Data:
- Real data (consumption, indoor temperature, weather) ensures accuracy and reliability of models.